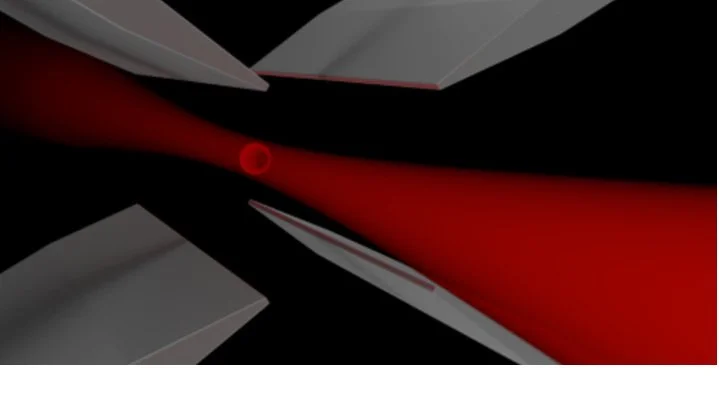
UCL Levitated Optomechanics: Quantum control and sensing on the nanoscale
Cooling and controlling the motion of levitated nanoparticles
We create nanomechanical oscillators by levitating nanoparticles using optical and electric fields. By trapping these particles in high vacuum, they are isolated from the environment allowing them to be cooled into their quantum groundstate. To cool these oscillators we use both feedback and cavity cooling to reduce their motional temperatures to the microKelvin regime. Not only do we cool their translation motion, but we have recently cooled both their rotational motion and their translational motion as well. We are part of the Atomic, Molecular, Optical and Positron Physics group at UCL.
Quantum mechanics in the macroscopic regime
Levitated nanomechaical oscillators are a brand new, large mass, quantum system that we are exploring. Quantum mechanics is largely untested on this scale and there is a significant worldwide push to create quantum or non-classical states of motion. This includes centre-of-mass superpositions. The relatively large mass of these quantum systems makes them the ideal test bed for investigating the quantumness of gravity in the lab.
Levitated quantum sensors
A staggering 85% of the universe's mass is dark matter, but we don’t yet know what dark matter actually is. Huge international efforts are now underway to find the answer to this important mystery. Our team is developing a new type of quantum sensor that uses the levitation of silica nanosphere spheres in vacuum. This sensor aims to detect the tiny collisions between the dark matter around us and the spheres. We collaborate with Chamkaur Ghag in the high energy physics (HEP) group at UCL and are part of the Cosmoparticle initiative.
News
The group headed to the Norwich Science Festival for two days with our outreach demonstration.
2025
Congratulations to Hayden and Fiona for submitting their PhD thesis.
The group partook at the Quantum Day celebration at Imperial College London as part of the International year of Quantum celebrations.
Congratulations to Amy for the new paper in the New Journal of Physics on A levitated atom-nanosphere hybrid quantum system Hopper and P F Barker 2024 New J. Phys. 26 013015 DOI 10.1088/1367-2630/ad19f6
We attended the UK Quantum Science Technology Showcase 2023 in the Business Design Center in Angel, London, demonstrating our levitated dark matter quantum sensor outreach setup to various stakeholders.
We sent Antonio off after an incredible 7 years in our group with a joint group BBQ dinner at Tania’s. - To new adventures in Florence and so long, Antonio.
We had our first joint KCL-UCL optotea group meeting, which will become a regular exchange to overcome common problems jointly in the levitation community.
We welcome Felipe Almeida as a new PhD student in the group. Bohdan Glisevic and Yuhang Zhai join for final year projects.
Congratulations to Markus who has just submitted his thesis! He will continue to work within the group on a Quantum Technology Fellowship.
Peter, Markus, Jon and Kelvin present our work to the public at Royal Society’s Summer Science Exhibition.
2024
The group headed to the National Quantum Technologies Showcase as part of the Quantum Technologies for Fundamental Physics (QTFP) exhibitor stand. Our exhibit included our demonstration Paul trap which levitates nanoparticles via electrostatic interactions.
We welcome Holly Owens to the group as research assistant and new PhD student starting February 2025!
Peter travels 1.2 km below the ground to visit the Boulby Dark Matter lab.
2023
Congratulations to Jonathan who submitted his PhD thesis shortly after we had our Christmas dinner.
Meet the Team
-
Peter Barker
Primary Investigator, Professor of Physics
-
Tania Monteiro
Primary Investigator, Professor of Physics
-
Jon Gosling
Postdoctoral Fellow
-
Markus Rademacher
Postdoctoral Fellow
-
Fiona Alder
PhD student
-
Julian Iacoponi
PhD student
-
Peiyao Xiong
PhD student
-
Hayden Fu
PhD student
-
Felipe Almeida Da Silva
PhD student
-
Eva Kilian
Postdoctoral Fellow
-
Holly Owens
Research Assistant
-
James Sabin
Research Assistant
Previous Members
-
Antonio Pontin
Postdoctoral Researcher - postdoctoral researcher CNR-INO, Italy
-
Kelvin Ho
Research Assistant - PhD student at the University of Innsbruck, Austria
-
Bohdan Glisevic
MSci Student - PhD student at ETH Zurich, Switzerland
-
Yuhang (Oliver) Zhai
Msci Student - MSc student at the University of Rochester, USA
-
Amy Hopper
PhD Student
2024
Optimal superpositions for particle detection via quantum phase
-
Exploiting quantum mechanics for sensing offers unprecedented possibilities. State-of-the-art proposals for novel quantum sensors often rely on the creation of large superpositions and generally detect a field. However, what is the optimal superposition size for detecting an incident particle from a specific direction? This question is nontrivial as, in general, this incident particle will scatter off with varied momenta, imparting varied recoils to the sensor, resulting in decoherence rather than a well-defined measurable phase. By considering scattering interactions of directional particulate environments with a system in a quantum superposition, we find that there is an optimal superposition size for measuring particles via a relative phase. As a consequence of the anisotropy of the environment, we observe a feature in the limiting behavior of the real and imaginary parts of the system’s density matrix, linking the optimality of the superposition size to the wavelength of the scatterer. https://doi.org/10.1103/PhysRevResearch.6.023037
-
Near-field, radially symmetric optical potentials supported by a levitated nanosphere can be used for sympathetic cooling and for creating a bound nanosphere-atom system analogous to a large molecule. We demonstrate that the long range, Coulomb-like potential produced by a single blue detuned field increases the collisional cross-section by eight orders of magnitude, allowing fast sympathetic cooling of a trapped nanosphere to microKelvin temperatures using cold atoms. By using two optical fields to create a combination of repulsive and attractive potentials, we demonstrate that a cold atom can be bound to a nanosphere creating a new levitated hybrid quantum system suitable for exploring quantum mechanics with massive particles. https://doi.org/10.1088/1367-2630/ad19f6
2023
Dark Matter Searches with Levitated Sensors
-
Motivated by the current interest in employing quantum sensors on Earth and in space to conduct searches for new physics, we provide a perspective on the suitability of large-mass levitated optomechanical systems for observing dark matter signatures. We discuss conservative approaches of recoil detection through spectral analysis of coherently scattered light, enhancements of directional effects due to crosscorrelation spectral densities, and the possibility of using quantum superpositions of mesoscopic test particles to measure rare events. https://doi.org/10.1116/5.0200916
-
Optomechanical devices are being harnessed as sensors of ultraweak forces for applications ranging from inertial sensing to the search for the elusive dark matter. For the latter, there is a focus on detection of either higher energy single recoils or ultralight, narrow-band sources; a directional signal is expected. However, the possibility of searching for a stochastic stream of weak impulses, or more generally a directional broadband signal, need not be excluded; with this and other applications in mind, we apply Gaussian white noise impulses with a well defined direction to a levitated nanosphere trapped and 3D cooled in an optical tweezer. We find that cross-correlation power spectra offer a calibration-free distinctive signature of the presence of a directional broadband force and its orientation quadrant, unlike normal power spectral densities (PSDs). We obtain excellent agreement between theoretical and experimental results. With calibration we are able to measure the angle , akin to a force compass in a plane. We discuss prospects for extending this technique into the quantum regime and compare the expected behavior of quantum baths and classical baths. https://doi.org/10.1103/PhysRevResearch.6.013129
Controlling mode orientations and frequencies in levitated cavity optomechanics
-
Cavity optomechanics offers quantum ground state cooling, control and measurement of small mechanical oscillators. However optomechanical backactions disturb the oscillator motions: they shift mechanical frequencies and, for a levitated oscillator, rotate the spatial orientation of the mechanical modes. This introduces added imprecisions when sensing the orientation of an external force. For a nanoparticle trapped in a tweezer in a cavity populated only by coherently scattered (CS) photons, we investigate experimentally mode orientation, via the Sxy (ω) mechanical cross-correlation spectra, as a function of the nanoparticle position in the cavity standing wave. We show that the CS field rotates the mechanical modes in the opposite direction to the cavity backaction, canceling the effect of the latter. It also opposes optical spring effects on the frequencies. We demonstrate a cancellation point, where it becomes possible to lock the modes near their unperturbed orientations and frequencies, independent of key experimental parameters, while retaining strong light-matter couplings that permit ground state cooling. This opens the way to sensing of directionality of very weak external forces, near quantum regimes.
Sympathetic cooling and squeezing of two colevitated nanoparticles
-
Levitated particles are an ideal tool for measuring weak forces and investigating quantum mechanics in macroscopic objects. Arrays of two or more of these particles have been suggested for improving force sensitivity and entangling macroscopic objects. In this article, two charged, silica nanoparticles, that are coupled through their mutual Coulomb repulsion, are trapped in a Paul trap, and the individual masses and charges of both particles are characterized. We demonstrate sympathetic cooling of one nanoparticle coupled via the Coulomb interaction to the second nanoparticle to which feedback cooling is directly applied. We also implement sympathetic squeezing through a similar process showing nonthermal motional states can be transferred by the Coulomb interaction. This work establishes protocols to cool and manipulate arrays of nanoparticles for sensing and minimizing the effect of optical heating in future experiments. https://doi.org/10.1103/PhysRevResearch.5.013070
Simultaneous cavity cooling of all six degrees of freedom of a levitated nanoparticle
-
Controlling the motional degrees of isolated, single nanoparticles trapped within optical fields in a high vacuum are seen as ideal candidates for exploring the limits of quantum mechanics in a new mass regime. These systems are also massive enough to be considered for future laboratory tests of the quantum nature of gravity. Recently, the translational motion of trapped particles has been cooled to microkelvin temperatures, but controlling all the observable degrees of freedom, including their orientational motion, remains an important goal. Here we report the control and cooling of all the translational and rotational degrees of freedom of a nanoparticle trapped in an optical tweezer, accomplished by cavity cooling via coherent elliptic scattering. We reached temperatures in the range of hundreds of microkelvins for the translational modes and temperatures as low as 5 mK for the librational degrees of freedom. This work brings within reach applications in quantum science and the study of single isolated nanoparticles via imaging and diffractive methods, free of interference from a substrate. https://doi.org/10.1038/s41567-023-02006-6
Measurement of the motional heating of a levitated nanoparticle by thermal light
-
We report on measurements of the photon-induced heating of silica nanospheres levitated in a vacuum by a thermal light source formed by a superluminescent diode. Heating of the nanospheres motion along the three trap axes was measured as a function of gas pressure for two particle sizes and recoil heating was shown to dominate other heating mechanisms due to relative intensity noise and beam pointing fluctuations. Heating rates were also compared with the much lower reheating of the same sphere when levitated by a laser. https://doi.org/10.1103/PhysRevA.107.013521
Recent publications
Sympathetic cooling and squeezing of two co-levitated nanoparticles
-
Levitated particles are an ideal tool for measuring weak forces and investigating quantum mechanics in macroscopic objects. Arrays of two or more of these particles have been suggested for improving force sensitivity and entangling macroscopic objects. In this article, two charged, silica nanoparticles, that are coupled through their mutual Coulomb repulsion, are trapped in a Paul trap, and the individual masses and charges of both particles are characterized. We demonstrate sympathetic cooling of one nanoparticle coupled via the Coulomb interaction to the second nanoparticle to which feedback cooling is directly applied. We also implement sympathetic squeezing through a similar process showing nonthermal motional states can be transferred by the Coulomb interaction.
Levitodynamic spectroscopy for single nanoparticle characterization
-
Fast detection and characterization of single nanoparticles such as viruses, airborne aerosols and colloidal particles are considered to be particularly important for medical applications, material science and atmospheric physics. In particular, non-intrusive optical characterization, which can be carried out in isolation from other particles, and without the deleterious effects of a substrate or solvent, is seen to be particularly important. Optical characterization via the scattering of light does not require complicated sample preparation and can in principle be carried out in-situ. We describe the characterization of single nanoparticle shape based on the measurement of their rotational and oscillatory motion when optically levitated within vacuum. Using colloidally grown yttrium lithium fluoride nanocrystals of different sizes, trapped in a single-beam optical tweezer, we demonstrate the utility of this method which is in good agreement with simulations of the dynamics. Size differences as small as a few nanometers could be resolved using this technique offering a new optical spectroscopic tool for non-contact characterization of single nanoparticles in the absence of a substrate.
https://doi.org/10.48550/arXiv.2401.11551